Gerhard Dobler and Sergey Tkachev
Key Points
- Tick-borne encephalitis virus (TBEV) exists in natural foci, which are areas where TBEV is circulating among its vectors (ticks of different species and genera) and reservoir hosts (usually rodents and small mammals).
- Based on phylogenetic studies, four TBEV subtypes (Far-Eastern, Siberian, European, Baikalian) and two putative subtypes (Himalayan and “178-79” group) are known. Within each subtype, some genetic lineages are described.
- The European subtype (TBEV-EU) (formerly known also as the “Western subtype”) of TBEV is prevalent in Europe, but it was also isolated in Western and Eastern Siberia in Russia and South Korea.
- The Far-Eastern subtype (TBEV-FE) was preferably found in the territory of the far-eastern part of Eurasia, but some strains were isolated in other regions of Eurasia.
- The Siberian (TBEV-SIB) subtype is the most common and has been found in almost all TBEV habitat areas.
- The Baikalian subtype is prevalent around Lake Baikal and was isolated several times from ticks and rodents.
- In addition to the four TBEV subtypes, one single isolate of TBEV (178-79) and two genetic sequences (Himalayan) supposed to be new TBEV subtypes were described in Eastern Siberia and China.
- The data on TBEV seroprevalence in humans and animals can serve as an indication for the presence or absence of TBEV in studied area.
The natural focus
In the early 1920s, reports surfaced concerning a severe form of brain disease in woodcutters, topographers, road construction workers, and residents of newly founded villages in the Taiga forest in the far eastern region of the former Soviet Union. The severity of the disease was such that in 1937 an expedition was organized to detect the origin of this unusual disease. During this first Taiga expedition to identify the etiology of a newly occurring form of encephalitis, Zil’ber et al.1 showed that the etiologic agent of this disease seemed to be a filterable pathogen that was transmitted by ticks of the genera Ixodes and Dermacentor. In at least 2 more expeditions to study the transmission of this disease (later named Russian Spring Summer Encephalitis, and currently known as tick-borne encephalitis [TBE]) Pavlovsky recognized that it was associated with specific types of landscape, and from this observation he developed his theory on the nature of human diseases.2
In his theory, Pavlovsky describes a natural focus (“Nidus”) of a disease as an area where specific climate, vegetation, soil, and favorable microclimatic conditions exist, so that vectors, donors, and recipients of infection find favorable conditions to exist. In this respect a natural focus of disease is related to a specific geographical landscape. According to this theory, humans acquire a zoonosis with natural foci only if they are in the territory of the natural focus in a definite season of the year and if they are attacked as prey by hungry vectors or come into contact with the animal reservoir (via hunting), which have already acquired the infection as carriers or donors of the respective agent.
During the last century a number of scientists, especially from Russia and the Czech Republic, studied in detail the landscapes that are associated with the occurrence of TBE. Rosicky3 and Blaskovic4 defined landscape types of TBE natural foci (Figure 1).
Figure 1: Different landscape types of TBE natural foci (according to Rosicky3 and Blaskovic et al.4)
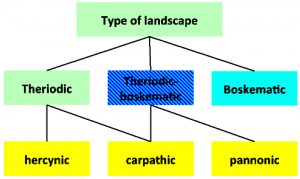
According to this classification, a theriodic focus is a focus in a forest with game animals as the main vertebrate hosts for adult ticks. A boskematic focus is a focus where meadows dominate and where farm animals are the main vertebrate hosts for adult tick stages. The theriodic-boskematic form is a mix of the two, having both types of landscape.
Another classification was made by Blaskovic et al.,4 who categorized the natural foci according to their main geographic location into Hercynian foci (located mainly in the Central German Uplands), Carpathian foci (located in the far southeastern part of Europe), and Pannonian foci (located at the western part of the Hungarian Danube lowlands). Similarly, Korenberg et al.5 made a classification according to the main geographic type (and not so much landscape type) for the TBE foci in Eurasia (Figure 2).
Figure 2: Eurasian TBE focus regions classified after Korenberg et al.5
(1) Central European-Mediterranean; (2) Eastern European; (3) Western Siberian; (4) Central Siberian-Trans-Baikalian; (5) Khingan-Amur; (6) Pacific; (7) Krim-Caucasian; (8) Kazakh-Central Asian

Click the image above to enlarge
By these classifications, the European TBE foci are located in the Central European–Mediterranean TBE focus region according to Korenberg et al.5 The classification developed by Rosicky3 indicates the European TBE foci are mainly of the theriodic type, while Eastern European countries have the mixed type or rarely also the boskematic type. Overall, these classifications may be helpful in getting an impression of the focus type in the landscape, but they are not very helpful for describing a TBE natural focus in detail. Also, so far, no clear associations have been identified between genetic profiles or phenotypic characteristics of TBEV strains and their respective focus types.
The natural cycle
As described above, a natural focus is an area where the ecological conditions allow the presence and transmission of a pathogen. In the case of TBEV, a natural focus is an area where TBEV is circulating among its vectors (ticks of different species and genera) and vertebrates (usually rodents and small mammals, which support the transmission of the TBEV). Details of these transmission cycles and the animal species involved are described in Chapter 3. However, at the moment it is not clear which ecological structures and requirements are needed to establish and maintain a TBE natural focus. A sufficient number of ticks that are infected or might be susceptible to infection must be present. Also, a sufficient number of susceptible small mammals to support virus transmission is required. There must also be an adequate number of larger animals to support the developmental cycle of the nymphs and adult stages of the tick vectors, as these are rarely found on rodents. The virus itself is transmitted via viremic vertebrates or via co-feeding of TBEV-infected ticks together with non-infected ticks, with the latter transmission mechanism being more effective. However, so far, no proof exists as to the actual importance of any of these mechanisms in the field.
A number of models on natural foci of TBEV are now available, but fieldwork is missing. In the early 1960s Austrian researchers were studying TBE foci in Austria.6 According to the authors’ data and estimates, focus size was 60,000 m2 with an estimated 2 million larvae and about 500,000 nymphs in the focus. They estimated that between 500 and 1500 nymphs (0.1% to 0.3%) are infected at any time in the year and may infect 15 to 30 rodents out of an estimated total number of 700 rodents in the focus. They found a total of 4 small mammal species with a clear dominance of Apodemus spp. (Apodemus flavicollis > Apodemus sylvaticus > Myodes glareolus > Microtus agrestis). The focus was highly fragmented into old forests, young forests, and meadows that existed within the forests.
Nosek et al.7 described the structure of TBE natural foci in the Czech Republic. Their work showed that a focus is maintained by a number of so-called microfoci. The size of the natural focus is not given. The authors estimate that per 10,000 m2 (1 ha) the number of ticks ranges from 15,000 to 50,000 nymphs. A microfocus is defined as a structure in the focus area where virus transmission is continuously active and therefore the virus can be generally detected. The rate of positive ticks in the microfocus is approximately 0.5% to 1% in nymphs and up to 5% in adult ticks.
In a recent study over 4 years in a TBE focus in Hungary, the authors reported that an area of 36 ha (3,600,000 m2) was screened and that only in an area of 0.49 ha (4900 m2) seropositive rodents were detected.8 They found TBEV in a total of 3 tick pools (2 pools of Ixodes ricinus and 1 pool of Haemaphysalis concinna) out of 7,247 sampled ticks (0.05%). Of note, in an area around 170 m away from the focus but in the same natural focus area, no TBEV was detected among 2369 sampled ticks. This description supports our own observation on TBE natural foci in southeastern Germany9 that a TBE natural focus has a size of about 5000 to 10,000 m2. The main ecological structure, which can be identified as important in the focus, is the ecotone between forest and meadow. More data must be collected in the field to get a clear picture of the ecological structure that is required for the development and maintenance of a TBE natural focus.
Table 1: Seroprevalence of anti-TBE anti-bodies in normal populations of different European countries
Country | Prevalence (%) | Literature |
---|---|---|
Bornholm (Denmark) | 1.4 | Kristiansen17 |
Estonia | 0-5 | Vasilenko et al.72 |
Archipel (Finland) | 5 | Han et al.73 |
Lithuania | 3 | Juceviciene et al.74 |
Norway | 2.4 | Skapaas et al.75 |
Poland (North) | 4.8-6.5 | Anonymous 1983 |
Czech Republic | 15-28 | Gresikova 198876 |
Switzerland | 0.5-5.0 | Matile et al. 197977 |
Hunchun (China) | 10.9 | Satz 200678 |
The phylogeny and phylo-geography of TBEV
According to phylogenetic studies at least 3 and possibly 6 subtypes of the TBEV can be genetically distinguished by molecular technologies. At present, 3 subtypes of TBEV—the European (western) subtype (TBEV- EU), the Siberian subtype (TBEV-SIB), and the Far-Eastern subtype (TBEV-FE)—are recognized. Russian virologists have claimed 2 new subtypes, strain 178-19 and strain 886-84, both isolated in the Lake Baikal region in Siberia.10 Also, a new putative TBEV Himalayan subtype was claimed in China.84 The European subtype differs by 4% to 6% from the other 2 subtypes (amino acid sequence). The Siberian and Far-Eastern subtypes also differ by 4% to 6% in amino acid sequence from each other.
Phylogenetic analysis shows that the TBEV group separated from the other flaviviruses about 30,000 years ago in Central Africa. From there, the tick-borne flavivirus ancestors migrated east and arrived in central Siberia about 7,500 years ago. The virus ancestor then divided into a western branch and an eastern branch. The eastern branch developed into the Siberian and Far-Eastern subtypes plus also into potentially 2 newly identified subtypes. This evolutionary development took about 3,000 years. The western branch spread to Central Europe and further evolved on the British Isles into Louping ill virus and on the Iberian Peninsula into the Spanish sheep encephalitis virus.11
In Western Europe, TBEV-EU is prevalent. However, in the Baltic countries and in parts of Finland, the Siberian and Far-Eastern subtype virus strains have been isolated and identified. So far, it is not clear whether the Siberian subtype in particular moves in a western direction. However, identification of virus strains in Siberia shows that a few of the strains circulating in Siberia belong to the European and Far-Eastern subtypes. According to results from Russian investigators, the Siberian subtype invaded the Baltic countries only recently, coincidentally with the construction of the Trans-Siberian Highway and the Trans-Siberian Railway.12 Also, the European subtype has been detected in South Korea and also in Siberia.13,14 Improved understanding of the phylogeography of these strains will require additional studies.
Table 2: Seroprevalence of anti-TBE antibodies in high-risk populations of different European countries
Country | Risk group | Prevalence (%) | Literature |
---|---|---|---|
Bornholm (Denmark) | Forest worker | 16 | Kristiansen71 |
Germany | Forest worker | 5.6-7.2 | Satz78 |
Alsace (France) | Forest worker | 8 | Collard et al.79 |
Poland (North) | Forest worker | 20-40 | Satz78 |
Switzerland | Forest worker | 4.7 | Matile et al.77 |
Hungary | Forest worker | 3.3 | Molnar80 |
European subtype
The European subtype (formerly known as the “Western subtype”) of TBEV is prevalent in Europe. However, the distribution ranges from France and The Netherlands at its western limit of distribution to South Korea, the easternmost region where TBEV-EU has been detected so far.9,13,15 While only TBEV-EU is found in Central Europe, more than 80% of identified strains in the Baltics belong to the European subtype. In Western and Eastern Siberia, only a low percentage (<10%) of the identified TBEV strains is characterized as European subtype. As noted, some other TBEV-EU strains have been identified and isolated in South Korea.13,16,17
According to phylogenetic data, TBEV-EU is the youngest of all TBEV subtypes.11 These data indicate that about 3,000 years ago the European strain diverged from the ancestor virus and migrated westwards. Some evidence suggests that the TBEV strains in Central Europe originated in the Czech Republic. From there the virus migrated about 350 years ago to Germany.18 Several waves of spreading and migration seem to have occurred. In Germany intensive studies on particular TBE foci show that in each TBE focus, a particular and clearly identifiable virus strain is prevalent. The TBEV strains seem to be stable in their E gene sequences for decades as shown in Finland (Kumlinge strain) and in Austria (Zillertal strain).9 However, no clear pattern of viral spread exists that can be correlated to landscapes or to human activities to explain the introduction of the Siberian and Far-Eastern subtypes in the Baltic region. Analysis of the E genes of TBEVs from different strains shows a kind of geographic clustering e.g. in Scandinavia, Germany, the Czech Republic or the Slovak Republic (Slovakia). But there are also some strains that are genetically related to strains from greater distances, e.g. German strains that are similar to Russian or Scandinavian strains. It is unclear at the moment whether these genetic relationships are due to missing link strains. A clear classification of European strains into genetic clusters or branches is still missing and awaits the analysis of more strains from different parts of Europe.
The phylogenetic analysis of TBEV-EU is unclear and confusing. For about 3,000 years, when the European strain branched off from the ancestor virus and migrated westward, TBEV-EU appears to have remained mono-phylogenetic. All currently known strains from Central Europe separated only about 300 to 400 years ago.11 In contrast to the Siberian subtype, the European subtype shows a parallel evolution. All currently known strains seem to originate from a single genetic clade. In contrast, the Siberian subtype shows a more consecutive genetic evolution. Only recently, a TBEV strain from The Netherlands was shown to have a distant genomic relationship to all other TBEV-EU strains. While TBEV-EU has also been identified and isolated outside Europe, the phylogenetic connection between European strains and the Siberian and Korean strains is as yet unclear.
A number of phenotypic characterizations have demonstrated TBEV strains of differing pathogenicity, which are circulating in nature. The TBEV strain MucAr HB171/11 shows low neuropathogenicity and neuro-invasiveness in a mouse model.9 A Czech strain, ts263, is a temperature-sensitive strain that does not grow at 40°C and also exhibits non-neuro-invasiveness.19
In addition, TBEV-EU is mainly associated with the biphasic form of TBE. So far, no chronic forms of disease caused by TBEV-EU have been reported. The clinical picture of infection ranges from subclinical to febrile disease to CNS symptoms with severe and persisting neurological sequelae in up to 10% of human cases. The fatality rate of infections with TBEV-EU ranges from 1% to 2%. Acute fatal cases have been rare since a fast-acting treatment of brain edema was introduced. Disease sequelae and fatal cases are mainly seen in elderly patients. The fatalities often result from super-infections (e.g. pneumonia) relating to the neurological sequelae (e.g. paralysis of breathing muscles); therefore these conditions must be named as indirect causes of fatalities due to TBE.
Far-Eastern subtype
The TBEV-FE viral subtype can be primarily found in the territory of the far-eastern part of Eurasia.20-27 However, this subtype was detected in other regions of Eurasia, including the Baltic countries, the Crimean Peninsula, the Republic of Moldova, the Republic of Belarus, and the territories of Komi Republic, Republic of Bashkortostan, Ural Mountains, Siberia, and the European part of Russia.10,28-32 In some territories, TBEV-FE has been more prevalent in urban and suburban areas.33,34 Also, TBEV-FE can cause different forms of disease, from subclinical to acute.35,36
Within this subtype at least 4 separate groups (lineages) of TBEV have been described (Figure 3). The first group consists of TBEV strains similar to the Sofjin strain, which was isolated in the Khabarovsk region of Russia in 1937 from a patient’s brain (Zil’ber, 1939)1 and includes strains from far eastern Russia, Japan, China, Latvia, and the European part of Russia.26,27 The group of strains similar to the Oshima strains isolated in Japan on Hokkaido Island forms a separate cluster on phylogenetic dendrograms that is significantly different from the Sofjin strains group20-22 and includes TBEV strains from Japan, China, and the Crimean peninsula.26,27 The third group consists of the Chinese Senzhang strain, which was isolated from a patient’s brain in 195324; the MGJ-01 strain, which was obtained from a patient’s blood serum and used in China for the production of vaccines and immunobiologic drugs37; and other strains from far eastern Russia. In addition, the fourth group formed by TBEV-FE strains from Japan (Kam586/97(AB237185), Kam588/97(AB237186)) has been described.27 The time of divergence among different TBEV- FE clusters within the Far-Eastern subtype was estimated at approximately 470 to 650 years ago (Figure 3).
Also, within TBEV-EU some unique virus variants have been described. In 1999, in the southeast of the Novosibirsk region of Western Siberia, Russia, cases of hemorrhagic forms of TBE with fatal outcomes were reported.38 Previously, infections resulting in a hemorrhagic disease had not been described for TBEV, although other tick-borne flaviviruses such as Omsk hemorrhagic fever virus and Kyasanur forest disease virus may cause blood-clotting (see section 6 below). The sequencing of the E gene fragment of 6 samples (Figure 3) shows that these TBEV variants corresponded to TBEV-FE, and a number of observed nucleotide substitutions (and amino acid substitutions in the corresponding E protein fragment) were not previously described. Thus, the appearance of new variants of highly pathogenic, atypical TBEV can be evidence of the continuing evolution of this virus group.
In 2004, the TBEV Glubinnoe/2004 strain was isolated from the brain of a deceased patient in the Primorsky region of far eastern Russia. The sequencing of its genome demonstrated that this TBEV variant corresponds to TBEV-FE, but has 53 or 57 substitutions in polyprotein amino acid sequence compared with Far-Eastern strains 205 (DQ989336)39 or Sofjin-HO (AB062064),40 respectively, and 14 of these substitutions are unique and have not been described previously.41 Researchers also found that Glubinnoe/2004 has a high level of production of infectious viral particles during the early stages of infection in cell cultures as compared with other Far-Eastern 205 strains.41
Figure 3: The fragment of the TBEV dendrogram corresponding to TBEV-FE strains27
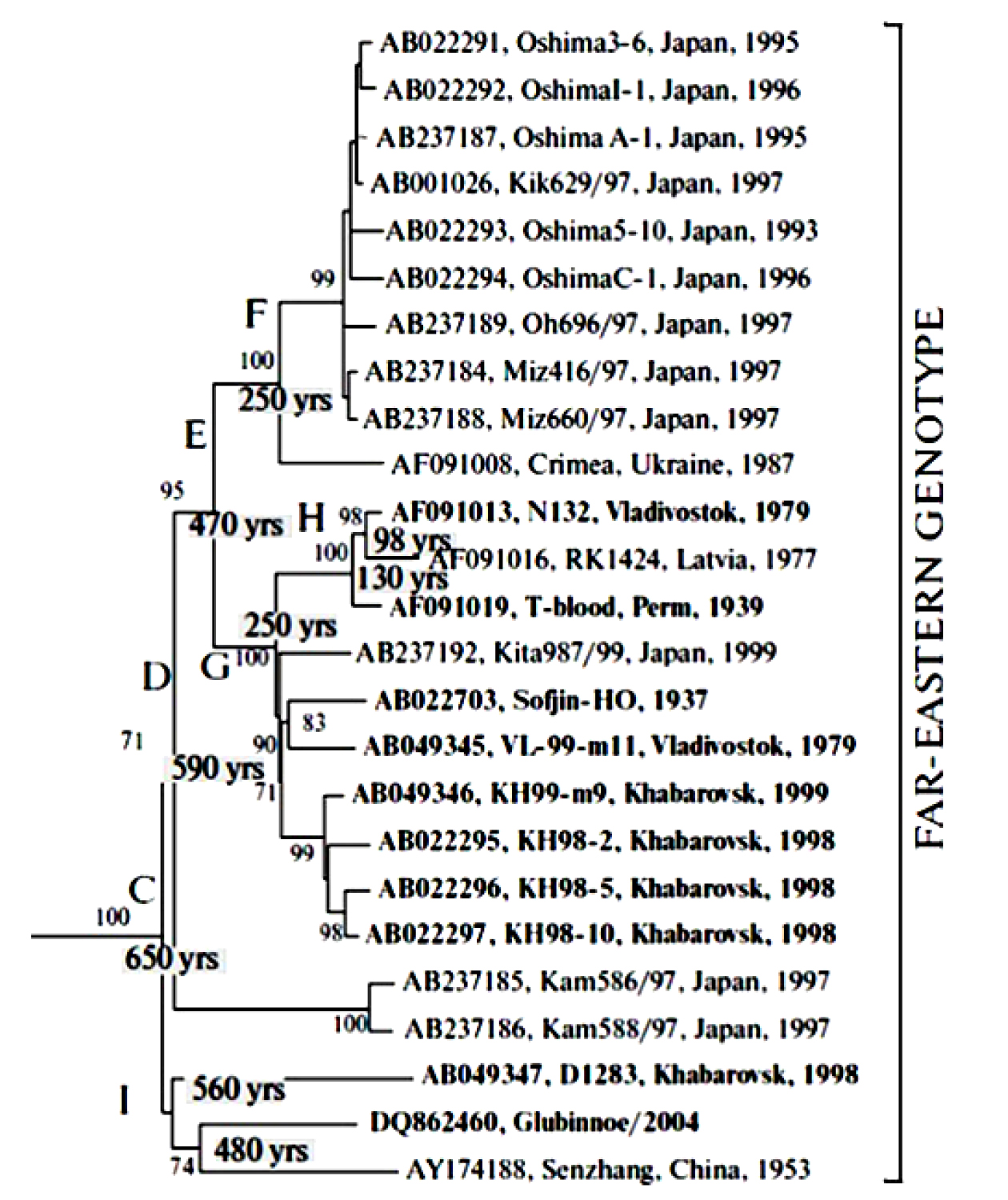
Click the image above to enlarge
Siberian subtype
The TBEV-SIB subtype is the most common TBEV and has been found almost everywhere in TBEV habitat areas. Thus, it has been detected in most parts of Russia, including the central and northwestern regions, Ural Mountains, Western and Eastern Siberia, the Far East, etc10,12,28,42-44as well as in Mongolia,45 Kazakhstan and Kyrgyzstan,46-49 Finland and the Baltic countries,12,50 Ukraine,28,49 and the Balkan peninsula.49
TBEV-SIB is believed to be the most genetically heterogeneous, with a nucleotide substitution level about 5.4% within the subtype.51 At first, based on the analysis of E protein sequences at amino acid positions 234 and 431, two genetic lineages were defined: one lineage including Zausaev strain (AF527415) was characterized by H234/ A431, whereas strains of the second lineage including Vasilchenko strain (AF069066) revealed Q234/ T431.52,53 Later, the “Baltic lineage”50,54–56 and “European topovariant”57 of TBEV-Sib were described. Also, the heterogenicity of TBEV-Sib was demonstrated by molecular hybridization of nucleic acids with 2 subgenotype-specific probes (designated as 3a and 3b) differentiating lineages/ subgenotypes “Vasilchenko” and “Zausaev” of Siberian subtype (Figure 4).10 The Zausaev and Vasilchenko lineages were found in various regions of Eurasia at different ratios, and moreover, some TBEV strains of Siberian subtype could not be attributed to any of these lineages.
Figure 4: Correlation and distribution of TBEV genotype 3 subgenotypes throughout the whole sampling area and Eastern Siberia. Altogether, 197 strains were typed using oligonucleotide probes10

Click the image above to enlarge
Baikalian subtype
In addition to the 3 primary and accepted TBEV subtypes, 2 groups of TBEV strains supposed to be new TBEV subtypes were described. At this time, the members of now accepted fourth prototype strain 886-84 (EF469662, KJ633033) subtype have been found only in the Republic of Buryatia, in the Irkutsk and Chita regions of Eastern Siberia and in northern Mongolia (Figure 5).10,21,51 This subtype is also now named “Baikalian subtype” and about 20 TBEV strains have been identified and genetically characterized.10,49,51 These strains (called the “886-84 group”) form an independent cluster on the TBEV dendrogram (see Chapter 2) and have no close homology with any strains of the 3 original subtypes. Within the group, high homology (more than 98%) of nucleotide sequences was observed while the genetic differences with other subtypes were shown to be greater than 12%.51
TBEV strains of the Baikalian subtype were isolated from ticks and small mammals collected in the Irkutsk region, Buryat Republic, and Transbaikalia in 1984-1990 indicating their ecological connection with all elements of the transmission chain. Despite the fact that these strains were isolated over 20 years ago, their circulation probably continues in natural foci. Thus, 2 TBEV strains similar to the reference strain of the Baikalian subtype were described recently in the territory of Transbaikalia from a taiga tick (in 1999) and 1 strain from Myodes rutilus (in 2010).58,59 Also, in 2010, a report was published on a case of fatal meningoencephalitis in Mongolia caused by a TBEV isolate having a high degree of homology in the E gene fragment (98.5%) with strains of the 886-84 group.60 The case was described in Bulganskiy province, bordering to the south with foci where TBEV group 886-84 strains had been isolated previously. The patient was hospitalized with meningoencephalitis on the 11th day after a tick bite and then died that same day. The presence of TBEV RNA in macromyelon samples, in the core and the meninx vasculosa, demonstrated the multilevel localization of lesions and was typical of the most severe forms of acute TBE that result in death or disability.60
The analysis of complete amino acid sequences of polyprotein from some strains confirmed that it is a “mixture” of sequences common for the 3 genotypes. Twenty-nine unique substitutions were detected that could probably be genotype-specific for group 886 members.51 The studies of biological properties demonstrated that group 886 strains have a wide spectrum of antigenic properties, hemagglutination and neutralizing activities, high virulence, and thermotolerance.
Other putative subtypes
Besides the now four accepted subtypes there are two genetically distant groups of viruses, which show high genetic distance to all known TBE virus strains. One virus was isolated only once. The prototype strain which is named “strain 178-79” (EF469661) and was isolated in 1979 from a tick pool of Ixodes persulcatus.10 The single available isolate and genome sequence show 10 to 16% difference to other TBEV subtypes on nucleotide level and 3 to 6% difference on amino acid level.10
Chinese researchers reported on another new TBEV subtype.84 Two TBEV sequences were detected in two specimens of Marmota himalayana, collected in the Haixi prefecture at an altitude of 2,994m in the Qinghai-Tibet Plateau in China. So far, no virus isolates are reported. Only the sequence of the complete genome and of the viral polyprotein have been available. According to these data, the virus differs in 16 to 18% on nucleotide level and in 6 to 8% on amino acid level from all other TBEV subtypes. According to a phylogenetic analysis the putative new subtype diverged earlier from the Far-eastern subtype than the Siberian subtype.
Seroepidemiology in humans
From the start of the use of antibody testing in this field, the prevalence rates of antibodies against TBEV (and other pathogens) were used to estimate the burden of infection as well as the burden of disease in human populations. Although these rates depend on a number of different factors (such as a person’s age, profession, leisure activities, place of living, interest in nature/outdoor activities, degree of protection measures, knowledge about disease and transmission, and vaccination status, as well as presence of cross-reacting viruses, assay technology used, etc.), the data at least serve as a rough indication for the presence or absence of TBE in an area.
In determining TBE seroprevalence rates, studies in the normal population have to be distinguished from studies and their results in highly exposed professionals such as woodcutters, farmers, or hunters. In European countries, the available seroprevalence rates in different countries in the normal population range from 0% to 39%. However, the highest of these values are usually found in special geographic conditions, for example 39% on Finnish islands in the Baltic Sea. Usually the seroprevalence rates in European populations range from 0% to 5% (Table 1).
While other studies on the prevalence rates in high-risk populations resulted in similar rates, some also indicated more extreme values under special conditions, e.g. >30% to 40% in some groups of forest workers in Poland (Table 2).
These data showed that the risk of acquiring TBE infection might be high, both in an exposed general population and in a high-risk population. However, many of these studies were conducted before the introduction of vaccines. Therefore, awareness of the disease among the general population in rural areas was low and personal protection measures usually were not applied. This might be one reason why in some areas the seroprevalence rates in the normal population might be in a similar range as seen in highly exposed groups.
Seroepidemiology in animals
Humans are not natural hosts of the TBEV. Therefore, the seroprevalence rates in humans usually give an incomplete picture of TBEV epidemiology. During the past few decades, a number of studies have been undertaken to study the seroprevalence rates in different species of wild and domestic animals. The seroprevalence rates of particular animals can document the presence of a transmission cycle. These data may also help with understanding the intensity of transmission in the natural cycle. In addition, they may document the role of particular animals in virus transmission and in the maintenance of the TBE transmission cycle. Recently, data on the prevalence of antibodies and virus were tested in wild and domestic animals to identify species that might be used as surrogates for detection of endemic areas.
The role of particular mice and voles, Apodemus flavicollis and Myodes glareolus, respectively, as primary vertebrate hosts for the virus in the transmission cycle was demonstrated in a number of isolations of virus strains in TBE natural foci and through experimental infections.61-63Also, Apodemus sylvaticus seems to support the transmission cycle as evidenced by high seroprevalence rates in Switzerland.64 In a recent study, Achazi et al.65 detected TBEV using molecular techniques in 6 rodent species in Germany: Apodemus agrarius, Apodemus flavicollis, Apodemus sylvaticus, Microtus arvalis, Microtus agrestis, and Myodes glareolus. The seroprevalence rates in rodents of different areas ranged from 0% to 72% (Table 3).
While the role of mice (Muridae) and voles (Cricetidae) for TBEV transmission seems clear, the importance of Insectivora is still not finally clarified. Different studies show that hedgehogs (Erinaceidae) are highly infested with ticks. Kozuch et al.62 detected up to 50% seroprevalence rates in hedgehogs in a study in Slovakia, and they could isolate a strain of TBEV from the hedgehog. Even less clear is the role of shrews (Soricidae). However, TBEV was isolated from a brain of a common shrew, Sorex araneus.66 According to early studies, the common mole (Talpa europaea) produces high viremia and therefore may act as a maintenance host in the natural transmission cycle. Systematic seroprevalence data on TBE antibodies in insectivores are not available.
In addition, seroprevalence studies in foxes and correlations with human TBE are limited. One study on TBEV seroprevalence in foxes from different areas in Germany found prevalence rates from 0% in Brandenburg to 10% in the Odenwald and Taunus region (a known endemic area of low activity) to 35% in the Black Forest area, a highly endemic region for TBE.67 Also a number of game animals have been tested as indicator animals for TBEV circulation.
These studies, in Germany but also in other European countries (e.g. Denmark), showed high seroprevalence rates against TBEV. Studies in Germany showed the seroprevalence rate in red deer and reindeer in the former German Democratic Republic was up to 72% positive.68 A similar rate of 83% was reported in a study from the Danish island of Bornholm, also in the red deer population.69 A study in red deer from Slovakia showed lower antibody rates of 35%.70
In natural transmission cycles of the boskematic type, the testing of antibody rates in farm animals may give good evidence of TBEV transmission and also of the risk of alimentary TBEV transmission. Therefore, a number a seroprevalence studies in cows, sheep, and goats from different countries are also available. In most available studies, these data show that the seroprevalence rate is around 5%. There are some exceptions in Germany. In the former German Democratic Republic, an antibody prevalence rate of 60% in cows was reported.68 A recent study in several federal states of Germany revealed seroprevalence rates of 0% to 43% in goats and sheep.85 The patchy distribution of high antibody rates in these animals correlated only in part with the presence of human TBE disease.
Other tick-borne mammalian flaviviruses
The International Committee on the Taxonomy of Viruses (ICTV) lists in the genus Flavivirus a total of eight tick-borne mammalian flavivirus (TBMF) species. (https://talk.ictvonline.org/ictv-reports/ictv_online_report/positive-sense-rna-viruses/w/flaviviridae). They distinguish single virus species according to several characteristics:
- Nucleotide and deduced amino acid sequence data.
- Antigenic characteristics.
- Geographic association.
- Vector association.
- Host association.
- Disease association.
- Ecological characteristics.
However, this actual species description no longer includes many of the known and ecologically different TBMF, as no virus subtypes or strains below species level are listed. However, there is a number of flaviviruses with specific names often found in literature, which cause severe human and animal disease. The known subtypes of TBMF are listed in Table 4 including some features regarding their geographical distribution and epidemiology.
All viruses listed are genetically closely related to the viruses of the TBEV complex. Therefore besides their medical and veterinary importance they also play a role regarding the diagnosis of flavivirus diseases due to cross-reactivity of antibodies with TBEV antibodies in areas of overlapping geographical distribution. For some of the viruses (Omsk hemorrhagic fever, Louping ill virus Kyasanur Forest disease virus) in laboratory tests the neutralizing cross-reaction of TBEV vaccine-induced antibodies was shown. However, no data are available on the field effectiveness of TBEV vaccines against these viruses.
Table 4: Viruses and virus subtypes of the tick-borne mammalian flavivirus complex of the tick-borne flavivirus group
Virus | Virus type/-subtype | Clinical symptoms in humans/in animals | Geographical distribution | Vector |
Louping ill virus | Louping ill virus
Meningoencephalitis Louping ill in sheep |
British Islands; possibly Norway | Ixodes ricinus | |
Turkish sheep encephalitis virus | No human disease known; encephalitis in sheep | Turkey | Unknown | |
Greek goat encephalitis virus | No human disease known; encephalitis is goats | Northern Greece | Ixodes ricinus | |
Spanish sheep encephalitis virus | No human disease known; encephalitis in sheep | Spain | Unknown | |
Spanish goat encephalitis virus | No human disease known | Northern Spain | Unknown | |
Negishi virus | Meningoencephalitis | Japan | Ixodes ricinus; Ixodes persulcatus | |
Omsk hemorrhagic fever virus | Omsk hemorrhagic fever virus | Hemorrhagic fever | Western Siberia | Ixodes apronophorus, Dermacentor spp. |
Kyasanur Forest virus | Kyasanur Forest virus | Hemorrhagic fever | Southwestern India; possibly China | Haemaphysalis spp. |
Alkhumra virus | Hemorrhagic fever | Arabian Peninsula; Egypt | Ornithodoros spp. | |
Powassan virus | Powassan virus | Meningoencephalitis | Northern America; Far east of Russia | Ixodes spp.; Dermacentor spp. (?) |
Deer tick virus | Meningoencephalitis | East coast of Northern America | Ixodes scapularis; Dermacentor andersoni | |
Langat virus | Langat virus | Meningoencephalitis in severly immuno-compromised patients | Malaysia to Central Siberia | Haemaphysalis spp. |
Acknowledgments
ST was supported by State funded budget project of ICBFM SB RAS #AAAA-A17-117020210027-9.
Contact:
Citation:
Dobler G, Tkachev S. General epidemiology of TBE. Chapter 11. In: Dobler G, Erber W, Bröker M, Schmitt HJ, eds. The TBE Book. 6th ed. Singapore: Global Health Press; 2023. doi: 10.33442/26613980_11-6
References
- Zil’ber LA. Spring (spring-summer) epidemic tick-borne encephalitis. Arch Biol Nauk. 1939;56:9-37.
- Pavlovsky EN. Natural Nidality of Transmissible Diseases. (Translated and edited by N.D. Levine). Urbana, IL: University of Illinois Press;
- Rosicky B. Notes on the classification of natural foci of tick- borne encephalitis in Central and South-East Europe. J Hyg Epidemiol Microbiol Immunol. 1959;3:431-43.
- Blaskovic D, Nosek J. The ecological approach to the study of tick-borne encephalitis. Prog Med Virol. 1972;14:275-320.
- Korenberg E, Kovalevskii YV. Main features of tick-borne encephalitis eco-epidemiology in Russia. Zentralbl 1999;289:525-39.
- Pretzmann G, Loew J, Radda A. Research on a natural focus of early summer meningoencephalitis (FSME) in lower Austria. 3. Attempt at a demonstration in toto of the cycle of FSME in the natural endemic focus. Zentralbl Bakteriol Orig. 1963;190:299-312.
- Nosek J, Kozuch O, Radda A. Studies of the ecology of the Central European tick-borne encephalitis virus in Northern Moravia. Zentralbl Bakteriol Orig. 1968;208:81-7.
- Zoldi V, Papp T, Rigo K, Farkas J, Egyed L. A 4-year study of a natural tick-borne encephalitis virus focus in Hungary, 2010- 2013. 2015;12:174-82.
- Dobler G, Gniel D, Petermann R, Pfeffer M. Epidemiology and distribution of tick-borne encephalitis. Wien Med Wochenschr. 2012;162:230-8.
- Demina TV, Dzhioev YP, Verkhozina MM, et al. Genotyping and characterization of the geographical distribution of tick-borne encephalitis virus variants with a set of molecular probes. J Med Virol. 2010;82:965-76.
- Heinze DM, Gould EA, Forrester NL. Revisiting the clinal concept of evolution and dispersal for the tick-borne flaviviruses by using phylogenetic and biogeographic analyses. J Virol. 2012;86:8663-71.
- Kovalev SY, Chernykh DN, Kokorev VS, Snitkovskaya TE, Romanenko VV. Origin and distribution of tick-borne encephalitis virus strains of the Siberian subtype in the Middle Urals, the north-west of Russia and the Baltic countries. J Gen Virol. 2009;90:2884-92.
- Kim SY, Yun SM, Han MG, et al. Isolation of tick-borne encephalitis viruses from wild rodents, South Korea. Vector Borne Zoonotic Dis. 2008;8:7-13.
- Demina TV, Tkachev SE, Kozlova IV, et al. Comparative analysis of complete genome sequences of European subtype tick-borne encephalitis virus strains isolated from Ixodes persulcatus ticks, long-tailed ground squirrel (Spermophilus undulatus), and human blood in the Asian part of Russia. Ticks Tick Borne Dis. 2017;8:547-53.
- Herpe B, Schuffenecker I, Pillot J, et al. Tick-borne encephalitis, southwestern France. Emerg Infect Dis. 2007;13:1114-6.
- Kim SY, Jeong YE, Yun SM, Lee IY, Han MG, Ju YR. Molecular evidence for tick-borne encephalitis virus in ticks in South Korea. Med Vet Entomol. 2009;23:15-20.
- Yun SM, Kim SY, Ju YR, Han MG, Jeong YE, Ryou J. First complete genomic characterization of two tick-borne encephalitis virus isolates obtained from wild rodents in South Korea. Virus Genes. 2011;42:307-16.
- Weidmann M, Ruzek D, Krivanec K, et al. Relation of genetic phylogeny and geographical distance of tick-borne encephalitis virus in central Europe. J Gen Virol. 2011;92:1906-16.
- Kopecky J, Krivanek K, Tomkova E. Attenuated temperature- sensitive mutants of tick-borne encephalitis (TBE) virus isolated from natural focus. In: Modern Acarology, Vol. 2. Eds Dusbábek F, Bukva V. The Hague: Academia, Prague and SPB Academic Publishing bv; 1991:11-9.
- Hayasaka D, Suzuki Y, Kariwa H, et al. Phylogenetic and virulence analysis of tick-borne encephalitis viruses from Japan and far-Eastern Russia. J Gen Virol. 1999;80 (Pt 12):3127-35.
- Zlobin VI, Demina TV, Mamaev LV, et al. Analysis of genetic variability of strains of tick-borne encephalitis virus by primary structure of a fragment of the membrane protein E gene. Vopr Virusol. 2001;46:12-6.
- Zlobin VI, Demina TV, Belikov SI, et al. Genetic typing of tick-borne encephalitis virus based on an analysis of the levels of homology of a membrane protein gene fragment. Vopr Virusol. 2001;46:17-22.
- Pogodina VV, Bochkova NG, Karan LS, et al. The Siberian and Far-Eastern subtypes of tick-borne encephalitis virus registered in Russia’s Asian regions: genetic and antigen characteristics of the strains. Vopr Virusol. 2004;49:20-5.
- Song H, Wang H, Wang P, et al. Design and application of M- RT-PCR diagnostic methods for arboviral encephalitis. J Microbiol Immunol. 2004;24:317–23.
- Leonova GN, Belikov SI, Pavlenko EV, Kulakova NV, Krylova NV. Biological and molecular genetic characteristics of a Far-Eastern tick-borne encephalitis virus population and its pathogenetic implication. Vopr Virusol. 2007;52:13-7.
- Si B-Y, Jiang T, Zhang Y, et al. Complete genome sequence analysis of tick-borne encephalitis viruses isolated in northeastern China. Arch Virol. 2011;156:1485-8.
- Subbotina EL, Loktev VB. Molecular evolution of the tick-borne encephalitis and Powassan viruses. Mol Biol (Mosk). 2012;46:82-92.
- Adel’shin RV, Zlobin VI, Belikov S, et al. Molecular epidemiology of tick-borne encephalitis in European part of Russia and some countries of Baltics, Eastern and South- Eastern Europe. Epidemiology and Vaccinal Prevention. 2006;2:27-3.
- Chausov EV, Ternovoi VA, Protopopova EV, et al. Variability of the tick-borne encephalitis virus genome in the 5′ noncoding region derived from ticks Ixodes persulcatus and Ixodes pavlovskyi in Western Siberia. Vector Borne Zoonotic Dis. 2010;10:365-75.
- Glushakova L, Korabel’nikov IV, Ternovoi VA, et al. Detection of causative agents in Ixodes persulcatus in the Komi Republic. Siberian Med J. 2012;111:88-91.
- Hubalek Z, Rudolf I. Tick-borne viruses in Europe. Parasitol Res. 2012;111:9-36.
- Ponomareva EP, Mikryukova TP, Gori AV, et al. Detection of Far-Eastern subtype of tick-borne encephalitis viral RNA in ticks collected in the Republic of Moldova. J Vector Borne Dis. 2015;52:334-6.
- Iurchenko OA, Vinograd NA, Dubina DA. Molecular genetic characteristics of tick-borne encephalitis virus in the Vopr Virusol. 2012;57:40-3.
- Mikryukova TP, Moskvitina NS, Kononova YV, et Surveillance of tick-borne encephalitis virus in wild birds and ticks in Tomsk city and its suburbs (Western Siberia). Ticks Tick Borne Dis. 2014;5:145-51.
- Tkachev SE, Fomenko NV, Rar VA, Igolkina YP, Kazakova YV, Chernousova NY. PCR-detection and molecular-genetic analysis of tick-transmitted pathogens in patients of Novosibirsk region, Russia. Int J Med Microbiol. 2008;298 (S1):365-7.
- Leonova GN, Belikov SI, Kondratov IG, Takashima Comprehensive assessment of the genetics and virulence of tick-borne encephalitis virus strains isolated from patients with inapparent and clinical forms of the infection in the Russian Far East. Virology. 2013;443:89-98.
- Lu Z, Broker M, Liang G. Tick-borne encephalitis in mainland China. Vector Borne Zoonotic Dis. 2008;8:713-20.
- Ternovoi VA, Kurzhukov GP, Sokolov YV, et al. Tick-borne encephalitis with hemorrhagic syndrome, Novosibirsk region, Russia, 1999. Emerg Infect Dis. 2003;9:743-6.
- Safronov PF, Netesov SV, Mikriukova TP, et al. Nucleotide sequence of genes and complete amino acid sequence of tick-borne encephalitis virus strain 205. Mol Gen Mikrobiol Virusol. 1991:23-9.
- Goto A, Hayasaka D, Yoshii K, Mizutani T, Kariwa H, Takashima I. Genetic and biological comparison of tick-borne encephalitis viruses from Hokkaido and far-eastern Russia. Jpn J Vet Res. 2002;49:297-307.
- Ternovoi VA, Protopopova EV, Chausov EV, et al. Novel variant of tickborne encephalitis virus, Russia. Emerg Infect Dis. 2007;13:1574-8.
- Bakhvalova VN, Rar VA, Tkachev SE, et al. Tick-borne encephalitis virus strains of Western Siberia. Virus Res. 2000;70:1-12.
- Pogodina VV. Monitoring of tick-borne encephalitis virus populations and etiological structure of morbidity over 60 years. Vopr Virusol. 2005;50:7-13.
- Tkachev SE, Demina TV, Dzhioev YP, et al. Genetic studies of tick-borne encephalitis virus strains from Western and Eastern Siberia. In: Flavivirus Encephalitis. Ed Růžek D: InTech; 2011:235-54.
- Frey S, Mossbrugger I, Altantuul D, et al. Isolation, preliminary characterization, and full-genome analyses of tick-borne encephalitis virus from Mongolia. Virus Genes. 2012;45:413- 25.
- L’Vov D K, Al’khovskii SV, Shchelkanov M, et al. Genetic characterisation of Powassan virus (POWV) isolated from Haemophysalis longicornis ticks in Primorye and two strains of Tick-borne encephalitis virus (TBEV) (Flaviviridae, Flavivirus): Alma-Arasan virus (AAV) isolated from Ixodes persulcatus ticks in Kazakhstan and Malyshevo virus isolated from Aedes vexans nipponii mosquitoes in Khabarovsk kray. Vopr Virusol. 2014;59:18-22.
- Abdiyeva K, Turebekov N, Shapiyeva Z, et al. The South of Kazakhstan is a hotspot for the Siberian Subtype of tick-borne encephalitis virus. 15th Medical Biodefense Conference Abstracts; 2016; Munich, 26-29 April, 2016:31.
- Hay J, Yeh KB, Dasgupta D, et al. Biosurveillance in Central Asia: Successes and Challenges of Tick-Borne Disease Research in Kazakhstan and Kyrgyzstan. Front Public Health. 2016;4:4.
- Tkachev SE, Tikunov AY, Babkin IV, et al. Occurrence and genetic variability of Kemerovo virus in Ixodes ticks from different regions of Western Siberia, Russia and Infect Genet Evol. 2017;47:56-63.
- Golovljova I, Vene S, Sjolander KB, Vasilenko V, Plyusnin A, Lundkvist A. Characterization of tick-borne encephalitis virus from Estonia. J Med Virol. 2004;74:580-8.
- Kozlova IV, Verkhzina MM, Demina TV, et al. Genetic and Biological Properties of Original TBEV Strains Group Circulating in Eastern Siberia. In: Ed Tkachev S: InTech; 2013:95-112. doi: 10.5772/54087
- Gritsun TS, Frolova TV, Pogodina VV, Lashkevich VA, Venugopal K, Gould EA. Nucleotide and deduced amino acid sequence of the envelope gene of the Vasilchenko strain of TBE virus; comparison with other flaviviruses. Virus Res. 1993;27:201-9.
- Gritsun TS, Frolova TV, Zhankov AI, et al. Characterization of a Siberian virus isolated from a patient with progressive chronic tick-borne encephalitis. J Virol. 2003;77:25-36.
- Mavtchoutko V, Vene S, Haglund M, et al. Characterization of tick-borne encephalitis virus from Latvia. J Med Virol. 2000;60:216-22.
- Golovljova I, Katargina O, Gellera J, et al. Unique signature amino acid substitution in Baltic tick-borne encephalitis virus (TBEV) strains within the Siberian TBEV subtype. Int J Med Microbiol. 2008;298:108-20.
- Tonteri E, Jaaskelainen AE, Tikkakoski T, et al. Tick-borne encephalitis virus in wild rodents in winter, Finland, 2008- 2009. Emerg Infect Dis. 2011;17:72-5.
- Karan LS, Pogodina VV, Frolova TV, Platonov AY. Genetic diversity of East European and Asian strains of tick-borne encephalitis virus belonging to Siberian genotype. Bull Siberian Med. 2006;S1:24-8.
- Andaev EI, Sidorova EA, Borisova TI, et al. Tick-borne encephalitis in the Trans-Baikal region and molecular-biological characteristics of the pathogen. National Priorities of Russia. 2011;2:148-50.
- Pogodina VV, Karan LS, Koliasnikova NM, et al. Polytypic strains in the gene pool of tick-borne encephalitis virus. Vopr Virusol. 2012;57:30-6.
- Khasnatinov MA, Danchinova GA, Kulakova NV, et al. Genetic characteristics of the causative agent of tick-borne encephalitis in Mongolia. Vopr Virusol. 2010;55:27-32.
- Ernek E, Kozuch O, Lichard M, Nosek J, Albrecht P. Experimental Infection of Clethrionomys Glareolus and Apodemus Flavicollis with Tick-Borne Encephalitis Virus. Acta Virol. 1963;7:434-6.
- Kozuch O, Gresikova M, Nosek J, Lichard M, Sekeyova M. The role of small rodents and hedgehogs in a natural focus of tick-borne encephalitis. Bull World Health Organ. 1967;36 Suppl:61-6.
- Radda A, Hofmann H, Pretzmann G. Threshold of viraemia in Apodemus flavicollis for infection of Ixodes ricinus with tick-borne encephalitis virus. Acta Virol. 1969;13:74-7.
- Burri C, Korva M, Bastic V, Knap N, Avsic-Zupanc T, Gern L. Serological evidence of tick-borne encephalitis virus infection in rodents captured at four sites in Switzerland. J Med Entomol. 2012;49:436-9.
- Achazi K, Ruzek D, Donoso-Mantke O, et al. Rodents as sentinels for the prevalence of tick-borne encephalitis Vector Borne Zoonotic Dis. 2011;11:641-7.
- Bardos V. Natural foci of neuro-infections in the Danube region. Cesk Epidemiol Mikrobiol Imunol. 1957;6:381-91.
- Rieger M, Nübling M, Müller W, Hasselhorn H-M. Foxes as indicators for TBE endemicity – a comparative epidemiological investigation. Zentralbl Bakteriol. 1999;289:610-8.
- Apitzch L. Untersuchung über Reservoire der Zeckenenzephalitis und ihre epidemiologische Bedeutung im Herdgebiet Torgelow. Zeitschr Ges Hyg. 1965;11:65-74.
- Freundt EA. The Western boundary of endemic tick-borne meningoencephalitis in southern Scandinavia. Acta Pathol Microbiol Scand. 1963;144:87-103.
- Labuda M, Eleckova E, Lockova M, A S. Tick-borne encephalitis virus foci in Slovakia. Int J Med Microbiol. 2002;291 suppl 33:43-7.
- Kristiansen K. TBE in Denmark – in particular on Bornholm. Int J Med Microbiol. 2002;291 suppl. 33:62-3.
- Vasilenko V, Golovljova I, Kutsar K, Jögiste A, Plyusnin A, A TBE foci in Estonia. Int J Med Microbiol. 2002;291, suppl. 33:182.
- Han X, Aho M, Vene S, et al. Studies on TBE epidemiology in Finland (and Lithuania). Int J Med Microbiol. 2002;291 suppl 33:48-9.
- Juceviciene A, Vapalahti O, Laiskonis A, Ceplikiene J, P Prevalence of tick-borne encephalitis antibodies in Lithuania. J Clin Virol. 2002;25:23-7.
- Skapaas T, Sundoy A, Bruu AL, et al. Tick-borne encephalitis in Norway. Tidsskr Nor Laegeforen. 2002;122:30-2.
- Gresikova M, Calisher CH. Tick-borne encephalitis. In: The Arboviruses: Epidemiology and Ecology. Ed Monath Boca Raton, Florida: CRC Press, Inc.; 1988:chapter 45, 177-202.
- Matile H, Aeschlimann A, R W. Seroepidemiological investigations on the incidence of TBE in man and dog in Switzerland. In: Tick-borne encephalitis. Ed Kunz C: International Symposium Baden/Vienna 1979, Facultas Verlag Wien; 1979:227-34.
- Satz N. Frühsommer-Meningenzephalitis (FSME). Bern, Switzerland: Verlag Hans Huber;
- Collard M, Gut JP, Christmann D, et al. L’encephalite a tiques en Alsace. Rev Neurol (Paris). 1993;149:198-201.
- Molnar E. Occurrance of tick-borne encephalitis and other arbovirus in Hungary. Geogr Medica. 1982;12:78-120.
- Han X, Aho M, Vene S, Peltomaa M, Vaheri A, Vapalahti Prevalence of tick-borne encephalitis virus in ticks in Finland. J Med Virol. 2001;64:21-8.
- Labuda M, Stützner D, Kozuch O, et al. Tick-borne encephalitis virus activity in Styria, Austria. Acta virol. 1993;37:187-90.
- Gresikova M, Masar I. Epidemiology of tick-borne encephalitis (TBE) in Czecho-Slovakia. Ellipse. 1991;29:451-3.
- Dai X, Shang G, Lu S, Yang J, Xu J. A new subtype of eastern tick-borne encephalitis virus discovered in Qinghai-Tibet Plateau, China. Emerg Microb Infect 2018;7:74.
- Klaus C, Beer M, Saier R, et.al. Goats and sheep as sentinels for tick-borne encephalitis (TBE) virus–epidemiological studies in areas endemic and non-endemic for TBE virus in Germany. Ticks Tick Borne Dis. 2012;3:27-37.